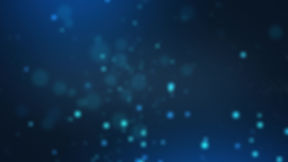
Nano Optics Group
Ariel
UNIVERSITY


RESEARCH
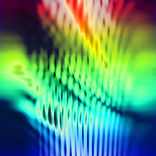
ACTIVE PLASMONICS
Through their unique dispersion relations, surface plasmon modes have provided new ways to modify and tailor light properties at the nanoscale. Enhanced light-matter interactions in light-confining structures (such as optical cavities) have been extensively investigated for both fundamental studies and practical applications. Plasmonic nanostructures, which can confine and manipulate light down to the nanometer scale, are becoming increasingly important. Such structures, for instance, can convert free-space light into intense, localized fields or enable coupling into deep-subwavelength-guided modes. Wherever subwavelength control over light is required, plasmonic structures are likely to play a vital role. Strong field enhancement in those structures can also alter light-matter interactions at a very fundamental level. Many areas of optical physics and devices can benefit from such extreme light concentration and manipulation. Moreover, these metal nanostructures can be simultaneously used as electrical contacts for current injection or application of electric fields. Thus, plasmonic structures are naturally suited for electrically driven devices. These active plasmonic devices can enable a broad range of new opto-electronic applications.
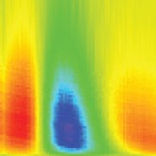
SINGULAR PLASMONICS
The energy flow within the electromagnetic field is often compared to fluid motion. Similarity of governing equations has led to discovery of such optical phenomena as optical Coriolis force, and optical Magnus effect.
In this context one can also think of an electromagnetic field with a phase, spiraling around some point just like water whirling around a sink outlet. In this singular point the phase is indefinite and the field amplitude is always zero while the energy flow encircles it. The field vorticity around the singular point can be calculated mathematically and even expressed in terms of an angular momentum of light. This angular momentum can be then transferred to real particles or to another electromagnetic field.
In recent years a novel branch of optics – nanophotonics – has emerged where the working length scales are nanometers (10e-9m). While classical optics is limited by a wavelength dimension, nanophotonics presents a new wave phenomenon – surface plasmon (SP) polaritons. This type of surface-confined electromagnetic radiation arises due to collective oscillations of bound charges at the surface of a metal and the characteristic length scale can be reduced beyond light's wavelength.
In this work we wish to investigate the interaction of structured (singular) light with the SP waves. The generation, transfer, modulation and absorption of the optical angular momenta can now be studied in two-dimensional geometry and in the subwavelength scales.

TIME RESOLVED LEAKAGE MICROSCOPY
Among the different surface plasmon imaging techniques, leakage radiation (LR) microscopy is a powerful method for imaging surface plasmon (SP) modes propagating on metal-dielectric interfaces. This imaging method has been implemented in a great variety of situations in SP optics, ranging from surface plasmon circuitry to near-feld weak measurements, both at the classical and quantum levels. Recently, this tech- nique has been combined with interference microscopy, providing not only the amplitude but also the phase of the leakage signal. We propose to operate LR microscopy in the time domain and demonstrate its efciency for performing ultrafast imaging of propagating SP wave packets at the diffraction limit. While our scheme leads to the si- multaneous measurement of both the group and the phase velocities of the SP wave packet, it also provides a unique method to resolve higher-order dispersive effects associated with the plasmonic signal, such as plasmonic group velocity dispersion effects. The simplicity of our all-optical method, that does not involve any raster-scanned local probe nor non-linear detection processes, presents a clear advan- tage with respect to the sophistication of recently proposed near-feld pulse tracking techniques (such as phase-sensitive time-resolved photon scanning tunneling microscopy, time-resolved two-photon photoemission electron or pulse-tracking via far-feld SP scattering interference imaging).
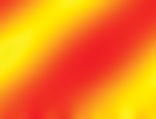
PLASMONIC METASURFACES
Metasurface can be regarded as a two-dimensional realization of metamaterial, i.e. a periodic or quazi-periodic structure made out of especially organized and accurately designed unit cells that exhibit a collective behaviour which is basically different from the bulk material from which the structure is made. Thus such a structure can be produces on an existing optical device, for instance a waveguide, providing it with a novel functionality. Chiral metasurfaces comprise of unit cells with lack of mirror symmetry. When properly designed, such structures can exhibit a peculiar effect of coupling of an intrinsic (spin) angular momentum of light to the extrinsic (orbital) angular momentum of surface plasmons – spin-orbit interaction (SOI). In analogy with a spinning top, that exhibits a precession when tilted, a light beam that fails to conserve its polarization helicity changes its propagation path. Recently, this effect was presented in order to directionally launch surface plasmons on a metal surface. We propose plasmonic metasurfaces that mediate a waveguide mode propagating inside a glass slab with a far-field radiation by using SOI. Accordingly the polarization of the light extracted by the metasurface carries a specific circular polarization that corresponds to the mode propagation direction. Contrarily, the light of a specific circular polarization incident on the metasurface will launch a guided mode in a specific direction. These and other effects are studied using nanofabricated chiral plasmonic metasurfaces.